Unraveling the Mysteries of the Double Helix Structure: A Comprehensive Overview
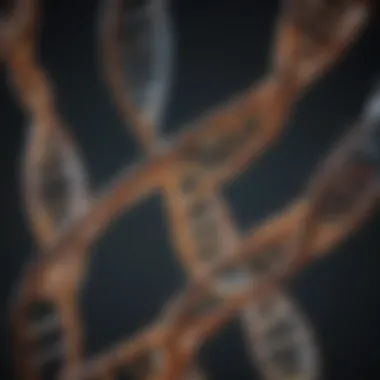
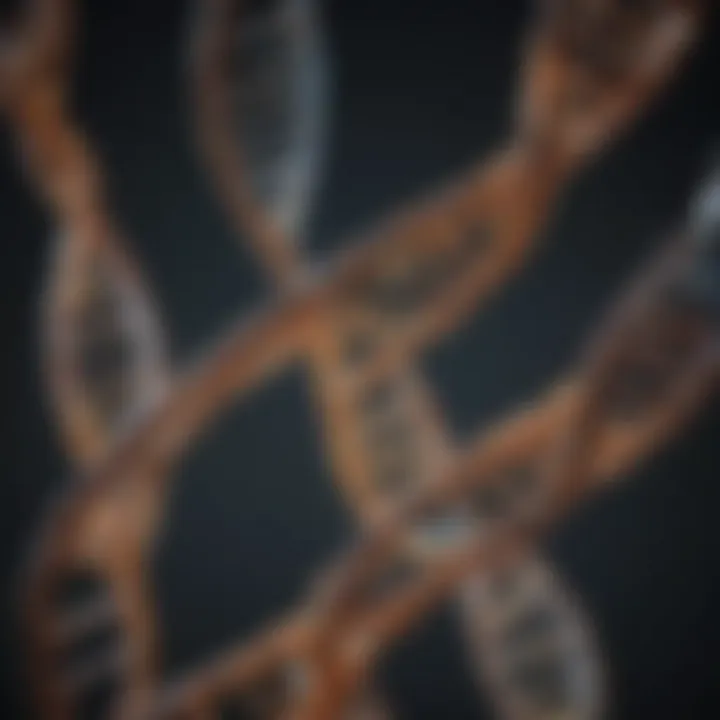
Book Title and Author Introduction
- The book unpacks the fundamental principles of the double helix structure, offering readers a comprehensive view of its components and functions. The author, with a background in molecular biology, brings a wealth of knowledge to the table, making this exploration both enlightening and enriching.
Summary of Book Content
- Within the pages of this enlightening publication, the intricate details of the double helix structure are carefully dissected. From the role of nucleotides to the significance of hydrogen bonds, every aspect is explored with precision and clarity. The book goes beyond surface-level explanations, delving deep into the genetic code embedded within the double helix, offering readers a profound understanding of its implications in scientific research.
- Highlighted quotes from the book encapsulate the essence of this comprehensive analysis. Phrases such as "The symphony of base pairs orchestrates life's genetic dance" serve as a poignant reminder of the complexity and beauty found within the double helix structure.
Three Lessons Learned
- Lesson 1: The first lesson extracted from the book emphasizes the importance of hydrogen bonds in stabilizing the double helix structure. By understanding the intricacies of these bonds, researchers can unlock new pathways for genetic exploration and manipulation.
- Lesson 2: Delving deeper, the second lesson highlights the role of nucleotide pairing in coding genetic information. Unraveling the intricate pairing mechanisms opens doors to deciphering the genetic code embedded within the double helix, paving the way for groundbreaking discoveries.
- Lesson 3: The final lesson explores the significance of protein synthesis in relation to the double helix structure. Understanding how genetic information is translated into functional proteins sheds light on the complexities of molecular biology and the vital role played by the double helix in this intricate process.
Practical Applications
- Real-life scenarios emerge where the lessons extracted from the book find practical application. From genetic engineering to pharmaceutical research, the insights gained from studying the double helix structure can revolutionize various scientific fields.
- Tips provided in the book offer guidance on implementing these lessons in daily research practices. By incorporating efficient nucleotide pairing techniques and leveraging hydrogen bonds effectively, researchers can enhance their understanding of genetic mechanisms and drive innovation in the field.
Introduction to the Double Helix
The Introduction to the Double Helix sets the groundwork for understanding the intricate world of genetics and molecular biology. In this article, we will delve into the historical context, significance in genetics, and the molecular biology perspective revolving around the double helix structure. Exploring these aspects will unveil the fundamental role that the double helix plays in shaping our understanding of genetic mechanisms and molecular processes. By dissecting each element, we aim to provide a comprehensive overview of the double helix, highlighting its critical importance in scientific research and discovery.
Historical Context
Initial Discoveries
The journey of the double helix begins with the Initial Discoveries that laid the foundation for our understanding of DNA's structure. These pivotal moments in scientific history marked the first glimpses into the intricate world of genetics. The Initial Discoveries not only unraveled the mystery of DNA but also paved the way for significant advancements in molecular biology. Their unique approach to studying genetic material revolutionized the field, propelling researchers towards decoding the secrets held within the double helix. Despite the challenges and uncertainties faced during these early endeavors, the Initial Discoveries set the stage for a new era of genetic exploration, shaping the course of scientific progress.
Pioneering Scientists
Pioneering Scientists emerged as key figures in the exploration of the double helix, contributing invaluable insights to the field of genetics. Their relentless pursuit of knowledge and unwavering dedication to unraveling the mysteries of DNA propelled scientific advancements to unprecedented heights. The Pioneering Scientists not only elucidated complex genetic mechanisms but also inspired future generations of researchers to delve deeper into the realm of molecular biology. Their groundbreaking discoveries and innovative approaches continue to serve as a cornerstone for modern genetic research, showcasing the profound impact that these visionaries have had on shaping our understanding of the double helix.
Significance in Genetics
Fundamental Structure
The Fundamental Structure of the double helix embodies the essence of genetic integrity and hereditary information. Comprising intricate patterns of nucleotide sequences, the Fundamental Structure serves as a blueprint for genetic code transmission. Its composition forms the basis for DNA replication and the transmission of genetic traits across generations. Understanding the nuances of the Fundamental Structure provides profound insights into the mechanisms governing genetic inheritance, shedding light on the intricate interplay of nucleotide sequences within the double helix.
Genetic Encoding
Genetic Encoding plays a crucial role in translating genetic information into functional gene products. By deciphering the genetic code embedded within the double helix, researchers unveil the secrets of protein synthesis and cellular processes. The Genetic Encoding process dictates the synthesis of specific proteins based on the genetic information encoded in DNA. This intricate mechanism governs cellular functions, highlighting the indispensable role of Genetic Encoding in translating genetic instructions into tangible biological outcomes.
Molecular Biology Perspective
DNA Replication
DNA Replication stands at the core of genetic preservation and cellular reproduction. The meticulous process of DNA Replication ensures accurate transmission of genetic information during cell division. By unraveling the intricacies of DNA Replication, researchers gain insights into the mechanisms that safeguard genetic integrity and ensure continuity of genetic information. Understanding the nuances of DNA Replication sheds light on the fundamental processes that drive cell division and enable genetic stability across generations.
Protein Synthesis
Protein Synthesis represents a key biochemical process essential for cellular function and organismal development. The intricate dance of ribosomes, transfer RNA, and messenger RNA orchestrates the synthesis of proteins based on the genetic code embedded in DNA. Protein Synthesis plays a pivotal role in translating genetic information into functional proteins, guiding cellular processes and molecular interactions. Delving into the realm of Protein Synthesis unveils the intricate mechanisms that underpin gene expression and protein function, offering a glimpse into the molecular choreography orchestrated by the double helix.
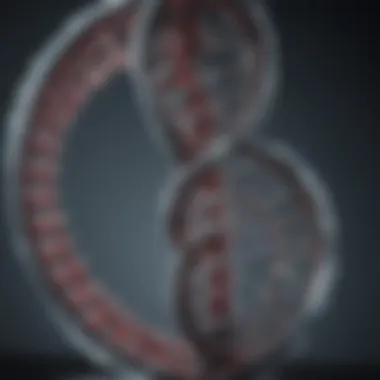
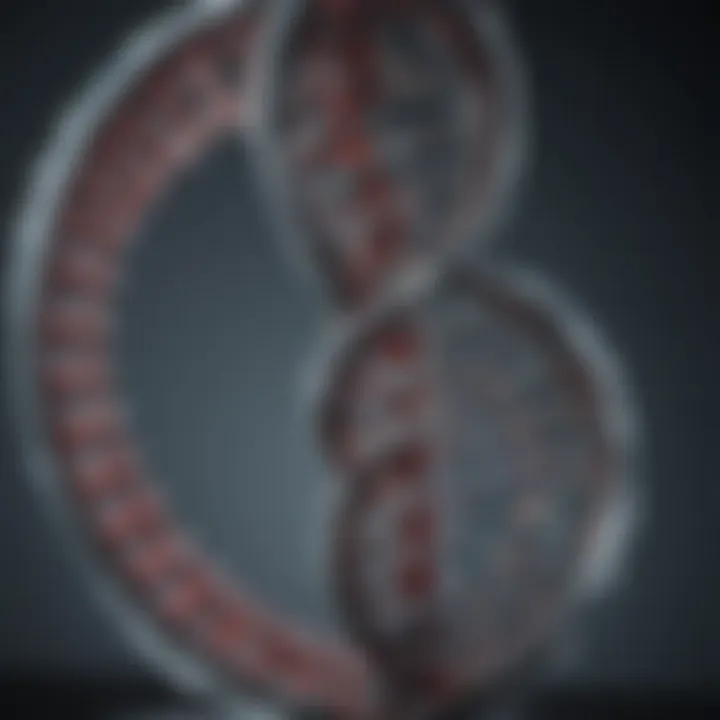
Understanding the Double Helix Structure
In this section, we delve into the critical aspects of Understanding the Double Helix Structure. Understanding the structure of the double helix is fundamental to grasp the intricacies of genetics and molecular biology. By exploring the arrangement of base pairs and the sugar-phosphate backbone, we gain valuable insights into the functioning of DNA at a molecular level. This understanding is pivotal in deciphering genetic information, unraveling DNA replication processes, and comprehending protein synthesis mechanisms essential for life processes.
Helical Arrangement
Base Pairs
Within the domain of molecular biology, base pairs are the cornerstone of DNA structure and function. Comprising adenine-thymine and guanine-cytosine bonds, base pairs play a vital role in genetic encoding and information transfer. The specificity of base pairing dictates the precise replication of DNA during cell division, ensuring the faithful transmission of genetic material. Their complementary nature enables accurate genetic coding, highlighting the significance of base pairs in preserving genetic integrity and hereditary traits.
Sugar-Phosphate Backbone
The sugar-phosphate backbone forms the structural framework that supports the base pairs in the DNA double helix. Its phosphate-sugar-phosphate linkage provides stability to the helical structure, safeguarding the genetic information contained within. This backbone not only offers structural support but also participates in molecular signaling and interactions essential for DNA functionality. The uniformity and flexibility of the sugar-phosphate backbone contribute to the overall stability and flexibility of the DNA molecule, facilitating essential biological processes with precision and efficiency.
Twisting and Coiling
Exploring the twisting and coiling of the double helix unveils the major and minor grooves, along with supercoiling dynamics. The major and minor grooves present distinctive surfaces for protein binding and molecular recognition, influencing gene expression regulation and DNA-protein interactions. Understanding the structural variations in these grooves offers insights into DNA-protein binding specificity and regulatory mechanisms crucial for genetic processes.
Supercoiling, on the other hand, involves the over- or under-winding of the DNA strands, impacting its compactness and accessibility to cellular machinery. This supramolecular arrangement plays a crucial role in gene regulation, chromatid condensation during cell division, and DNA packaging within the nucleus. The balance between supercoiling states determines the functional consequences on DNA transcription, replication, and repair processes.
Hydrogen Bonding
Base Pairing Rules
Hydrogen bonding governs the specificity of base pairing rules in DNA, enforcing the strict pairing between adenine-thymine and guanine-cytosine. These rules ensure the fidelity of genetic information transmission, preventing errors in DNA replication and transcription. The hydrogen bonds between complementary base pairs confer stability and specificity to DNA sequences, essential for maintaining the genetic code's integrity.
Stability Mechanisms
Stability mechanisms in DNA, including hydrogen bonding and base stacking interactions, contribute to the overall structural integrity of the double helix. These mechanisms counteract destabilizing forces, such as temperature fluctuations and external stress, maintaining the structural integrity and functional capacity of DNA molecules. By understanding these stability mechanisms, scientists can manipulate DNA structures for various biotechnological applications, targeting specific genetic sequences with precision and efficiency.
Functional Aspects of the Double Helix
In this section of our exploration on the double helix, we delve into the critical functional aspects that play a pivotal role in genetics and molecular biology. Understanding the functional elements of the double helix is paramount in grasping its significance in genetic information storage and gene expression. By dissecting the intricate mechanisms of the double helix's functionality, we shed light on how genetic material is stored, replicated, and expressed within living organisms.
Information Storage
Genetic Code
When dissecting the functional aspects of the double helix, the genetic code emerges as a central player in orchestrating the transfer of genetic information. The genetic code dictates how nucleotide triplets, known as codons, encode specific amino acids during protein synthesis. This process is fundamental in shaping an organism's characteristics and functions, highlighting the crucial role of the genetic code in molecular biology. Despite its complexity, the genetic code's universal nature across species underscores its intrinsic value in understanding genetic makeup and hereditary traits. However, variations in the genetic code can lead to genetic disorders or mutations, underscoring the delicate balance between stability and variability in genetic information transmission.
Mutations and Repair
Another key aspect of the functional dynamics of the double helix is the occurrence of mutations and the intricate repair mechanisms that organisms employ to maintain genetic integrity. Mutations serve as a double-edged sword, driving genetic diversity through evolutionary processes while posing risks of genetic diseases and abnormalities. Understanding how mutations arise and the mechanisms through which cells repair damaged DNA is crucial in comprehending the adaptive strategies organisms adopt in response to genomic insults. While mutations fuel genetic diversity, the precise balance between mutation rates and repair mechanisms is paramount to safeguarding genetic stability and ensuring the continuity of life processes.
Gene Expression
Transcription
Within the realm of gene expression, transcription stands as a vital process where genetic information encoded in DNA is transcribed into messenger RNA (m RNA) molecules. This intermediary step bridges the gap between gene sequences and protein synthesis, orchestrating the transfer of genetic instructions from the nucleus to the cytoplasm. Transcription not only elucidates the flow of genetic information but also regulates gene expression patterns, playing a fundamental role in cellular differentiation and specialization. The intricate regulation of transcription factors governs the precise activation and repression of genes, highlighting the sophisticated orchestration of gene expression in response to cellular demands.
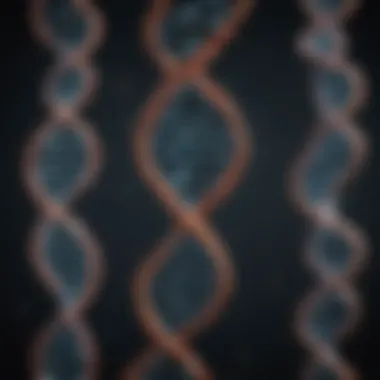
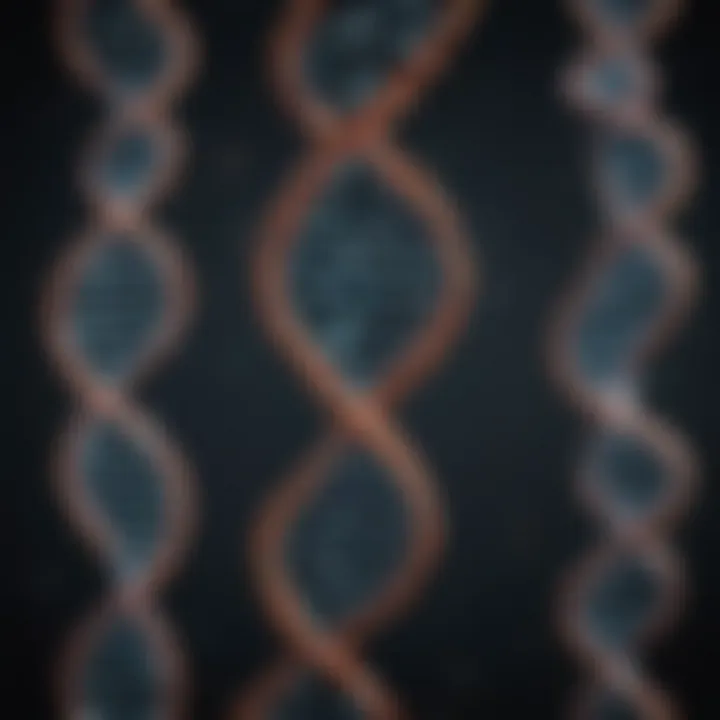
Translation
Complementing transcription, translation marks the culmination of gene expression, where m RNA is decoded to synthesize proteins at ribosomal complexes. This process involves the precise pairing of codons on mRNA with anticodons on transfer RNA (tRNA), ensuring the accurate assembly of amino acids into polypeptide chains. Translation is a highly coordinated process that underpins protein synthesis, dictating the structure and function of cellular proteins. The fidelity of translation mechanisms and the regulatory checkpoints involved underscore the stringent quality control measures cells employ to ensure protein functionality and integrity.
Regulatory Mechanisms
Epigenetics
In the realm of gene regulation, epigenetics emerges as a prominent regulatory mechanism that modulates gene expression without altering the underlying DNA sequence. Epigenetic modifications, such as DNA methylation and histone acetylation, play pivotal roles in shaping chromatin structure and influencing gene accessibility. These epigenetic marks serve as dynamic regulators of gene expression, orchestrating cellular responses to internal and external stimuli. The interplay between epigenetic modifications and transcriptional regulation highlights the intricate mechanisms organisms utilize to fine-tune gene expression patterns and phenotypic outcomes.
Gene Regulation
Concurrent with epigenetics, gene regulation governs the precise activation, repression, and timing of gene expression to maintain cellular homeostasis and functional specialization. Transcriptional regulators, enhancers, and silencers intricately modulate gene activity, shaping developmental processes and cellular responses. The combinatorial effect of gene regulatory networks not only underpins cellular identity but also influences adaptability and resilience in various environmental contexts. Understanding the coordination between epigenetic modifications and gene regulatory elements offers insights into the remarkable flexibility and robustness of cellular processes.
Applications and Implications of the Double Helix
In this pivotal section of the article, we delve into the far-reaching impacts of the double helix structure on various scientific domains. The applications and implications of the double helix extend beyond the realms of genetics and molecular biology, permeating into crucial areas of medical advancements, forensic science, and biotechnological innovations. By scrutinizing the double helix's structure and functionality, we uncover a myriad of possibilities for advancing healthcare, solving crimes, and revolutionizing biotechnology.
Medical Advancements
Genetic Testing:
Genetic testing plays a paramount role in deciphering an individual's genetic makeup, facilitating the diagnosis of genetic disorders, and predicting susceptibility to certain diseases. This technique allows healthcare professionals to tailor personalized treatment plans based on precise genetic information, promoting more effective and targeted interventions. Genetic testing offers insights into an individual's risk factors, enabling proactive health management and early detection of hereditary conditions. However, ethical considerations regarding genetic privacy and the potential psychological impact of test results warrant careful attention within the medical community.
Gene Therapy:
Gene therapy presents a groundbreaking approach to treating genetic disorders by introducing functional genes into patients' cells to replace or correct defective ones. By harnessing the power of genetic engineering, gene therapy holds immense promise for curing previously incurable genetic diseases and offering long-term solutions for patients. Despite its transformative potential, challenges such as immune responses, off-target effects, and ethical concerns surrounding genetic manipulation necessitate ongoing research and rigorous safety protocols. As gene therapy continues to evolve, its integration into mainstream medical practices promises a paradigm shift in healthcare delivery and disease management.
Forensic Science
DNA Profiling:
DNA profiling, also known as DNA fingerprinting, revolutionized forensic investigations by providing a unique genetic blueprint for identifying individuals with unprecedented accuracy. This forensic tool has been instrumental in solving criminal cases, exonerating the innocent, and establishing biological relationships in paternity disputes. The reliability and specificity of DNA profiling have reshaped the landscape of forensic science, offering irrefutable evidence that transcends traditional investigative methods. However, concerns regarding sample contamination, interpretation errors, and privacy issues underscore the need for stringent quality control measures and adherence to ethical guidelines.
Crime Investigations:
Crime investigations benefit significantly from the integration of DNA analysis techniques, enabling law enforcement agencies to link suspects to crime scenes, identify unidentified victims, and reconstruct timelines of events. DNA evidence serves as a compelling investigative tool, strengthening legal proceedings and enhancing the accuracy of criminal convictions. Despite its instrumental role in criminal justice, challenges related to forensic protocol standardization, evidence handling procedures, and courtroom admissibility persist, underscoring the importance of continuous training and technological advancements in forensic science.
Biotechnological Innovations
Recombinant DNA Technology:
Recombinant DNA technology revolutionized the field of biotechnology by enabling the modification of genetic material to produce novel organisms, proteins, and pharmaceuticals. This technique involves the insertion of foreign DNA fragments into host organisms, facilitating the expression of desired traits for research, industrial, and medical purposes. From developing genetically modified crops to producing recombinant insulin for diabetes treatment, recombinant DNA technology showcases the vast potential of genetic engineering in addressing societal challenges and advancing scientific innovation. However, ethical concerns regarding environmental impacts, genetic diversity, and biosafety regulations necessitate a balanced approach to harnessing the full potential of this technology.
Gene Editing:
Gene editing represents a cutting-edge tool for precise modification of genetic sequences within living organisms, holding immense therapeutic potential for treating genetic diseases and enhancing agricultural productivity. The advent of CRISPR-Cas9 technology has revolutionized gene editing capabilities, offering unprecedented precision, scalability, and versatility in manipulating DNA sequences. The applications of gene editing span diverse fields, from correcting disease-causing mutations to creating genetically modified organisms with desired traits. However, ethical debates surrounding germline editing, unintended genetic consequences, and equitable access to gene editing therapies underscore the importance of regulatory frameworks and ethical deliberations in guiding responsible use and dissemination of this transformative technology.
Future Perspectives on the Double Helix
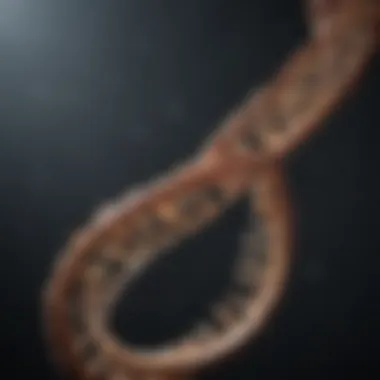
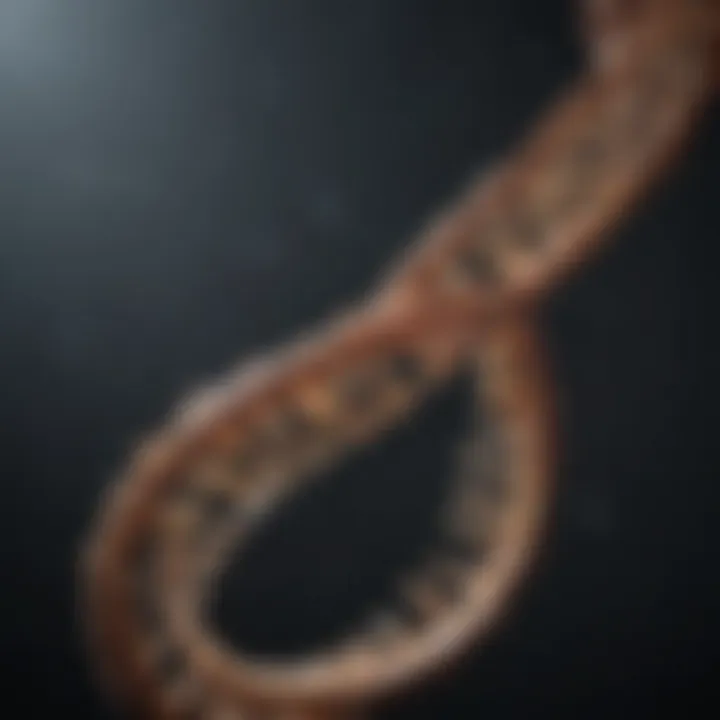
The section on Future Perspectives on the Double Helix aims to provide insights into the evolving trends and considerations in genetic research. Highlighting the advancements and challenges faced in the field, this section offers a glimpse into the potential trajectory of genetic studies.
Emerging Trends
CRISPR Technology:
Exploring CRISPR Technology unveils a groundbreaking approach to genetic editing. Its precision in targeting specific genes has revolutionized genetic research, offering immense potential for gene therapy and disease eradication. The versatility of CRISPR technology lies in its ability to edit DNA sequences accurately, acting as a powerful tool for genetic modification.
Synthetic Biology:
The realm of Synthetic Biology delves into the design and construction of biological parts, systems, and devices to mimic natural biological processes. Its role in advancing genetic engineering and biotechnology is significant, paving the way for creating novel biological entities with enhanced functionalities and characteristics. Embracing synthetic biology opens new avenues for creating sustainable solutions and bio-inspired technologies.
Ethical Considerations
Genetic Privacy:
Delving into Genetic Privacy sheds light on the ethical implications of genetic information sharing. The safeguarding of individuals' genetic data is paramount to ensure privacy and prevent misuse. Balancing the benefits of genetic research with the risks of potential privacy breaches is a critical consideration, urging for robust ethical frameworks to protect genetic privacy.
Designer Babies:
The concept of Designer Babies sparks debates on the ethical boundaries of genetic manipulation. Tailoring the genetic makeup of offspring for desired traits raises ethical concerns regarding natural selection, inequality, and unforeseen consequences. Exploring the realm of designer babies prompts a reevaluation of the ethical norms governing genetic enhancement.
Scientific Developments
Genome Editing:
Genome Editing emerges as a transformative tool in genetic modification, allowing precise alterations in DNA sequences. Its potential in correcting genetic defects and enhancing desired traits holds promise for medical treatments and agricultural improvements. The ethical implications of genome editing underscore the need for responsible use and regulation to navigate its impact on ecosystems and societies.
Precision Medicine:
Precision Medicine revolutionizes healthcare by tailoring treatments to individual genetic profiles. Its personalized approach considers genetic variations to optimize therapeutic outcomes, marking a shift from conventional medicine. Embracing precision medicine signifies a paradigm shift towards more effective and targeted medical interventions, reshaping the landscape of healthcare delivery.
Conclusion
The Conclusion section encapsulates the essence of the article, underlining the paramount significance of summarizing key points and elucidating the broader implications of understanding the double helix structure. By explicating the core elements discussed throughout the article, the Conclusion serves as a pivotal juncture summarizing the crucial takeaways for readers. Through a meticulous exploration of the double helix, this section solidifies the foundational knowledge presented in the preceding segments.
Summary of Key Points
Structure Importance
Investigating the nobility of Structure Importance, we unravel its pivotal role in illuminating the fundamental essence of the double helix. The inherent characteristic of Structure Importance lies in its ability to define the essence and resilience of genetic structures, offering a key foundation for comprehending genetic processes. In the intricate tapestry of this article, Structure Importance emerges as a beacon, guiding readers towards a profound comprehension of genetic architecture and molecular intricacies. Its unique ability to elucidate the core genetic framework exemplifies why Structure Importance stands as the quintessential cornerstone in deciphering the double helix puzzle.
Functional Significance
Delving into the depths of Functional Significance unveils its paramount contributions towards unravelling the mysteries of genetic functionality within the double helix. The crux of Functional Significance lies in its ability to decode the operational relevance of genetic information, shedding light on the intricate mechanisms governing genetic expression. As a critical component in the realm of genetics, Functional Significance symbolizes a linchpin linking theoretical understanding to practical genetic applications. The innate significance of Functional Significance lies in its capacity to bridge the conceptual gap between genetic theory and pragmatic genetic utilization, embodying a critical facet underscored in this article.
Final Remarks
Ongoing Research
The realm of Ongoing Research stands as a beacon of continuous advancement, propelling the understanding of the double helix into uncharted territories. By emphasizing the dynamic nature and evolving landscape of genetic research, Ongoing Research encapsulates the spirit of perpetual exploration and discovery within the genetic domain. The standout feature of Ongoing Research lies in its ability to push the boundaries of genetic knowledge, paving the way for groundbreaking revelations and transformative insights. Amidst the scientific panorama, Ongoing Research remains steadfast in its pursuit of unlocking new genetic frontiers, underscoring an indispensable element weaving through the fabric of this article.
Potential Applications
Pioneering into the potential applications of the double helix unfurls a realm of boundless possibilities, sculpting a narrative of innovation and progress within the genetic landscape. The crux of Potential Applications lies in its capacity to translate theoretical genetic understanding into real-world advancements, harnessing the genetic blueprint for diverse fields ranging from medical science to biotechnological innovations. As a pivotal conduit bridging theoretical genetic knowledge to tangible societal impacts, Potential Applications encapsulate the essence of progress and symbiotic evolution in the genetic domain. Against the backdrop of burgeoning technological advancements, the incorporation of Potential Applications unveils a kaleidoscopic vision of the transformative role played by genetic insights, solidifying its status as a cornerstone discussed within this article.